Passivhaus Trust - Why Choose MVHR for Higher Performing Buildings
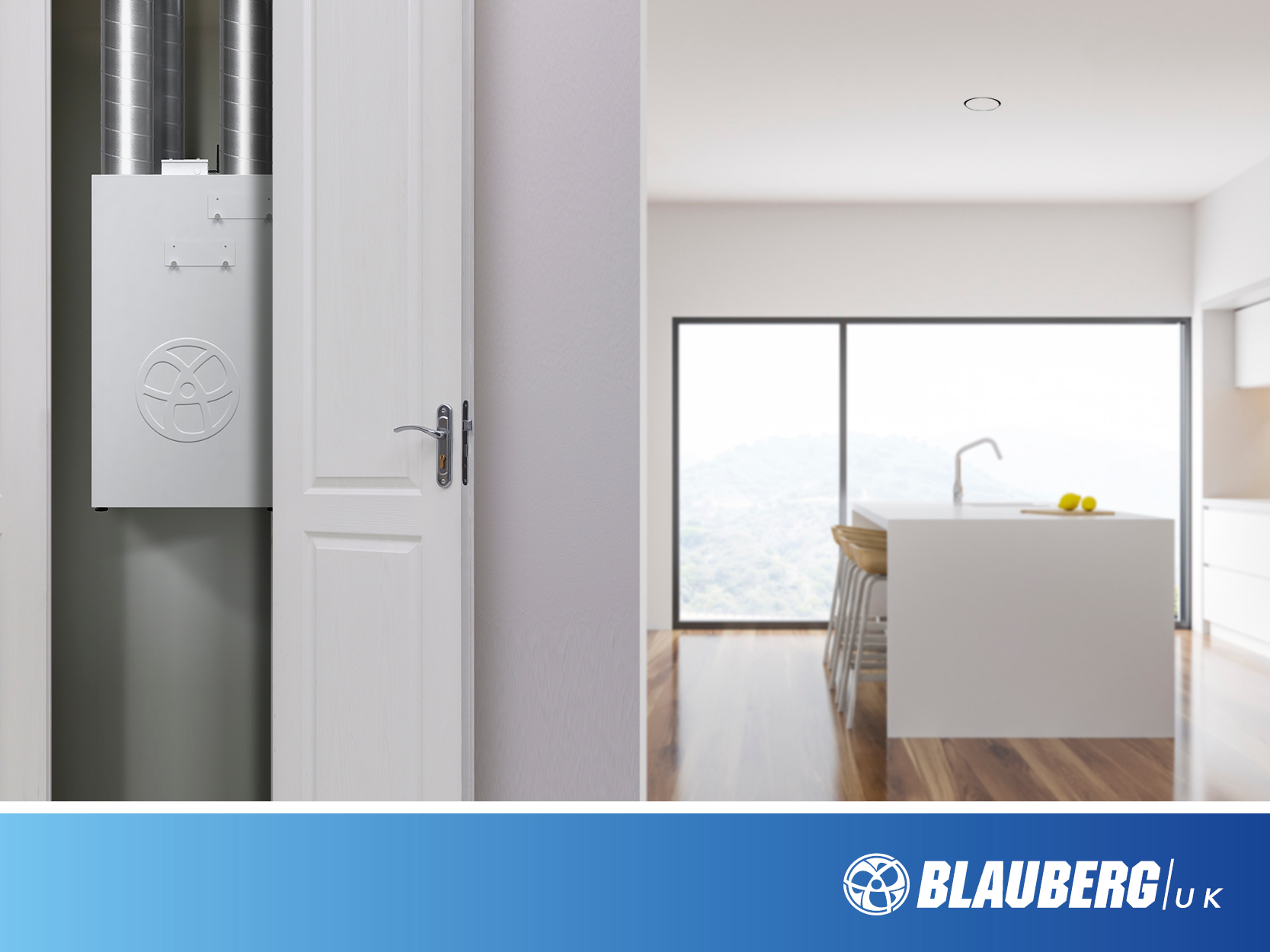
Introduction
Mechanical Ventilation and Heat Recovery (MVHR) systems are one of the ventilation options in domestic dwellings in the UK. They extract warm but potentially stale and humid air from bathrooms, kitchens, and utility rooms before passing this over a heat exchanger which transfers the heat to fresh air from outside the building.
These systems are more costly and more extensive than alternative ventilation mechanisms. There is also a widely adopted rule-of-thumb that MVHR systems are only effective in more airtight buildings, although this may in fact be incorrect. As a result, MVHR systems remain relatively niche in the UK with most dwellings being fitted with trickle ventilation and single room extract fans. In particular, MVHR systems are rarely fitted in retrofits unless particular attention has been paid to improving air-tightness.
This blog seeks to challenge this convention and suggests that MVHR systems are, in fact, beneficial in nearly all domestic situations, either new-build or retrofit.
So Why Ventilate?

Ventilation is needed in a building to remove the accumulation of indoor pollutants. These can enter from outside (e.g. particulates, pollen, radon, NOx and SOx fumes) or can be generated within the building (e.g. particulates from cooking, Volatile Organic Compounds (VOCs) from cleaning products and furniture, dust, humidity). The predominant factor in a domestic environment tends to be humidity and modelling suggests an air change rate of between 0.3 and 0.4 ACH will be effective in dealing with most typical domestic environments. Other pollutants tend to be produced at lower concentrations and so will be dealt with effectively at an air change rate of between 0.3 and 0.4 ACH1. Natural ventilation relies on opening windows, wind and the stack effect backed up by intermittent extract fans in bathrooms and kitchens. Wind speeds are variable and opening windows relies on the occupants. There is good evidence to show that this arrangement simply does not deliver appropriate levels of ventilation2. People are not inclined to regularly open windows in winter and intermittent extract fans are unable to deliver anywhere near 0.3 – 0.4 ACH.
They also do not run for long enough to deal with residual moisture from, for example, showering. All air entering the property is effectively uncontrolled and unfiltered, so external pollutants are drawn in from outside. Internal pollutants will only be dealt with if they are in a room with an extract fan, or if an occupant opens a window.
Mechanical Extract Ventilation (MEV), whilst able to provide a good level of fresh air, draws this air from the outside, through gaps in the fabric and trickle vents. Thus, the air is not filtered, and external pollutants are actually sucked into the building. There is also no real control over where air enters the building, so good levels of air exchange cannot be guaranteed in all rooms or areas. The stack effect means that vents or open windows in upstairs bedrooms may become an exhaust route for stale air rather than inlets for fresh.
An MVHR system will run continuously and thus should always achieve the required air change rate. In addition, because it is a balanced system, all the air entering the building (other than unwanted infiltration or when the occupant chooses to open windows) passes through the MVHR system and through a filter. Thus, a significant proportion of outdoor pollutants (particulates, pollen etc) are prevented from entering the building. The net result is that, regardless of the heat recovery element, an MVHR system will ensure higher levels of indoor air quality than is delivered by natural ventilation or MEV.
The table below summarises the characteristics of each ventilation strategy.

When is an MVHR System Effective?
If designed, installed and operated correctly, MVHR systems deliver excellent guaranteed levels of indoor air quality. However, as they include a heat exchanger, their performance is often evaluated in terms of energy or carbon savings rather than in terms of air quality.
In a recent Building Services Engineering Research and Technology article, Crawley et al3 suggest that MVHR systems should only be considered for dwellings with an air permeability of 3 m³/m2.hr or less as, beyond this, there is an additional net carbon emission penalty arising from the use of the MVHR system. This has been the industry consensus for some time and is backed up by references to earlier works from Lowe4 and Banfill et al5.
The research derives its results from the UK Standard Assessment Procedure (SAP). It is therefore simple to reproduce these results for any building using SAP as a basis. Figure 1 shows the equivalent results for a 90m2 semi-detached property and shows similar characteristics, with a crossover point at 3 m³/m2.hr.
This characteristic has typically been attributed to the greater electrical load on the MVHR as infiltration increases, and as electricity has traditionally had a greater carbon intensity than gas (the assumed fuel source for space heating), the MVHR will result in disproportionally higher emissions at higher levels of permeability.

How to MVHR Systems Behave?
However, this theory does not appear to match with the way in which MVHR systems actually behave. MVHR systems will normally operate at a constant flow rate regardless of temperature. They may have humidity or CO2 sensors which trigger a boost mode for short periods, but temperature will only be used to trigger a post heater (or other heating system), not to change the flow rate of air through the MVHR. Thus, as infiltration increases, there should be no effect on the energy required to run the MVHR.
So, perhaps this characteristic is due to less heat being recovered by the MVHR. However, this also doesn’t make sense. As the rate of infiltration increases, more cold air enters the building, which is then brought up to the set point temperature by the building’s heating system. So, unless the heating system is failing to maintain the set point temperature, the MVHR will be recovering the same volume of air at the same temperature, and using the same amount of electrical power, for all levels of air permeability.
This means that the MVHR system will be recovering the same amount of energy, regardless of the air permeability. In dwellings with higher levels of air permeability, there will be higher ventilation losses and thus a higher space heating demand which will result in SAP indicating a higher level of emissions. However, the crucial point is that this should be the same whether natural ventilation or MVHR is being used – it is the building’s space heating system which will compensate for the infiltration losses.
Examining the SAP calculation methodology reveals that the difference in ventilation characteristics is related to the way in which infiltration is treated for each mode. For natural ventilation, the effective ventilation rate is calculated using the formula below when the infiltration rate is below one:

The formula for natural ventilation also completely removes any natural ventilation (opening windows) once the infiltration rate is above one. This is effectively trying to predict occupancy behaviour as a function of air-tightness without any clear rationale.
To illustrate the impact of this discrepancy, the effective infiltration rate, nv,eff has been plotted against varying infiltration rates for both natural ventilation and for a MVHR system with no heat recovery. Figure 2 below shows that at all levels of infiltration above zero, SAP will use a higher level of effective ventilation (and therefore heat loss) for MVHR.

Figure 2 - Effective ventilation rates for Natural Ventilation and for MVHR in SAP for different infiltration rates
It should also be remembered that infiltration is, at best, a rough approximation. It will vary constantly, depending on wind speed and wind direction and will occur in unplanned locations throughout the building. Any prediction of infiltration behaviour, particularly at higher levels of air permeability, is therefore likely to be inaccurate.
Altering the infiltration characteristic to reflect a more gradual increase may be a valid approximation, but there seems no reason why this wouldn’t also apply in the case of MVHR ventilation. If we consider that infiltration is a factor of windspeed and gaps in the fabric both of these are not related to the ventilation mechanism within the building.
To test this premise, the SAP calculation was modified to apply the same calculation for effective ventilation to both natural ventilation and MVHR, with no modification to the infiltration rate. The results, shown in Figure 3 below, reveal that ventilation using MVHR results in lower carbon emissions for all levels of air permeability.

Evaluation of Previous Research
Having established that the results observed by Crawley et al are based on a questionable infiltration calculation within the SAP model, let us now consider the earlier research which appears to corroborate their results.
Lowe7 concludes that MEV ventilation will result in lower emissions than MVHR systems of intermediate levels of performance at air permeability of greater than 3 m³/m².hr. However, he also notes that MVHR equipment which is ‘efficient by current standards’ is likely to outperform MEV at all levels of air permeability.
Lowe also notes that dwellings using natural ventilation with an air permeability of less than 8 m³/m².hr are almost certainly likely to lead to under-ventilation as occupants will not open windows and, as a result he does not evaluate the relative performance of natural ventilation below 5 m³/m².hour in comparison to MVHR or MEV. In effect, he considers that natural ventilation is inappropriate below 5 m³/m².hour.
Lowe’s earlier work8 shows a linear relationship between air permeability and net CO2 emissions for both MVHR and natural ventilation. This matches with the modified SAP modelling results shown above. However, Lowe’s analysis shows that natural ventilation results in lower net CO2 emissions for all levels of air permeability, even for an ‘efficient’ MVHR system. Lowe’s definition of an efficient MVHR system is one with a constant fan power of 30W and a heat exchanger efficiency of 80%. These values are now quite poor-compared with modern MVHR systems (see Table 2 below) and thus, the net carbon emissions for a modern MVHR system will be considerably lower, potentially resulting in the MVHR option having lower emissions for all levels of air-tightness.
The Banfill et al analysis does not use SAP modelling, but instead uses dynamic thermal modelling (IES). The analysis models the CO2 emissions from just a single test building which was initially built to 1930 specifications before being subject to thermal and air-tightness upgrades. The analysis concludes that air permeability must be below 3 m³/m².h before there is a saving in energy use, but that the disparity in CO2 factors for gas and electricity means that, even at this level of air-tightness, overall CO2 emissions are slightly increased.
However, there are some key assumptions in the research methodology which are inconsistent with how MVHR systems are now actually designed and specified.
First, the analysis uses the UK Building Regulations standard of 0.3 l/s.m² as the continuous flow rate for the MVHR system. Whilst the paper acknowledges that this should only be a boost rate, activated to control odours and humidity, no control system was modelled and so the MVHR is assumed to run continuously at this higher rate. This is equivalent to around 0.5 ACH. Good design (based on Passivhaus modelling) is now to model to around 0.33 ACH on average. Assuming a higher flow rate than is actually used in practice increases both associated ventilation losses and results in higher levels of electricity consumption.
Second, the analysis picks up on the Building Regulations' suggestion that MVHR flow rates can be adjusted (increased) as the air-tightness of the property decreases. Again, in practice, this is not the case and MVHR flow rate adjustments are generally only linked to humidity or CO2 levels. The impact of this incorrect assumption is that the analysis models that MVHR ventilation losses increase at high levels of permeability.
Third, the analysis uses two MVHR standards – minimum building standards and best practice standards. The principal conclusions referenced by Crawley et al relate to the minimum building standards, not the best practice standards which indicate that MVHR systems save energy below 5 m³/m².h. Furthermore, the heat recovery efficiency and specific fan power used in the best practice analysis are much poorer than what might now be considered best practice – see Table 2.
Finally, in determining the relative CO2 reductions, all the previous analyses used the prevailing grid carbon factors at the time which effectively meant that electrical energy resulted in more than twice the CO2 emissions compared to gas. Since then, the electricity grid has de-carbonised significantly which is reflected in the new carbon factors proposed in SAP 10.1, thus electricity is now ‘cleaner’ than gas. This means that, even with outdated values for heat exchanger efficiency and fan power, if the new carbon factors were applied, the same analysis would offer very different results.
It is also worth highlighting some key points and caveats from the analysis which should be considered alongside the results:
- Infiltration levels are determined using a rough ‘rule of thumb’ method which simply divides the tested air change rate at 50Pa by 20.
- A control strategy which was able to modify MVHR flow rate in response to factors such as external temperature, occupancy and relative humidity could reduce electricity consumption.
- An MVHR system will ensure a reliable air change rate throughout the year, whereas this cannot be guaranteed by a natural ventilation strategy.
MVHR Performance Characteristics
The performance characteristics and flow rates of the MVHR systems modelled will have a significant effect on modelling results and indeed actual performance. The table below compares the key specifications of the MVHR systems used in the SAP model and the two reference papers against a poor and good specification based on current technology.

The table demonstrates that the previous analyses are based, predominantly, on what are now considered poor MVHR specifications and have also all applied a significantly higher flow rate than is now used in practice. SAP modelling also includes correction factors to account for poor design, but these cannot be reduced below minimum levels and thus good design is not rewarded. It is therefore impossible to fully model a well-designed and installed MVHR system in SAP.
Revising the Analysis
Taking into account the discussion above, the analysis has been re-run using different parameters.
First, the carbon factors have been changed to match the proposed SAP 10.1 grid intensity value of 136g/kWh for electricity and 210g/kWh for gas. The results, shown in Figure 4, indicate that this change alone results in MVHR ventilation out-performing natural ventilation by a significantly larger margin than previously, at any infiltration level.

Second, improved MVHR performance characteristics, taken from the ‘2020 Good’ column of Table 2 have been applied, in conjunction with the SAP 10.1 carbon factors. These results, shown below, highlight that the MVHR performance outperforms natural ventilation by between 20 and 30% in terms of carbon emissions for all levels of air-tightness.

Conclusion
This paper has shown that previous analyses indicating that MVHR systems should only be installed in properties with an air permeability of 3 m³/m².hour @50Pa or less are based on outdated information and flawed assumptions using a modelling system which was never intended to be used to compare ventilation systems.
A modern MVHR system will result in significantly lower CO2 emissions at any reasonable level of air permeability.
However, an MVHR system’s primary function is ventilation and, in this capacity, it is far more effective at providing a good quality indoor air environment – regardless of external conditions – than natural ventilation.
There is no clear up-to-date evidence behind the rule of thumb that says MVHR is inappropriate for dwellings with air permeability above 3m³/m².hour @50Pa. This analysis has shown that MVHR systems result in improved ventilation and lower carbon emissions for all levels of air-tightness. There is a compelling case for MVHR systems to be fitted in all new dwellings and to be strongly encouraged in retrofits where significant reductions in energy demand are being targeted.
Acknowledgements
PHT Author: John Plamer - Passivhaus Trust